Auditory pathways¶
The primary and non-primary pathways¶
There is a collection of tracts that connects the cochlea to cortex, known as the ascending auditory pathways:
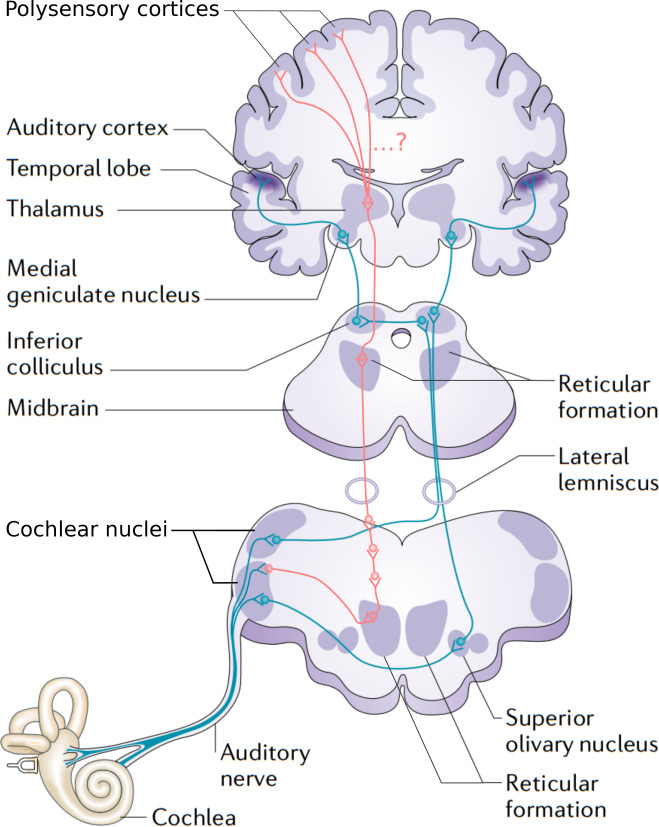
The primary auditory pathway is traced in blue, and is the one that we are most concerned with. The non-primary auditory pathway is traced in red.
The flow of information in this image is upward, from bottom to top, which is why the two pathways are “ascending”. The more precise anatomical term is afferent, which means ‘inward’ or ‘towards a central area’. In our particular case, it can mean ‘from sensory organs towards the brain’. By the way, the antonyms are ‘descending’ or efferent, as illustrated in the sidebar for a sensorimotor circuit.
Stating information flow in this pathway as exclusively ascending begs the question of whether realist or constructivist perception is correct, since no room is made for potential descending information. We will come back to this problem again and again.
Both pathways in The ascending auditory pathways 1 pass through the major subcortical areas, which are characterized by groups of neurons known as nuclei, the plural of nucleus:
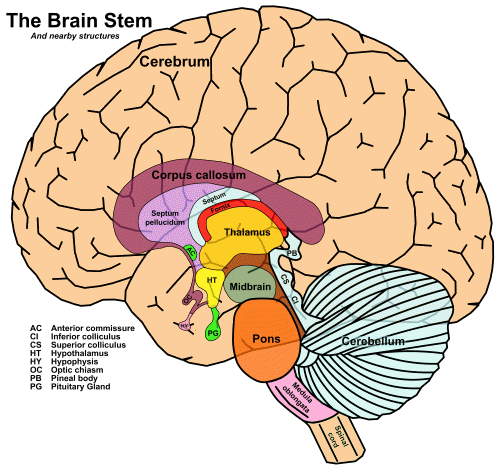
The four major structures, from bottom to top, are the medulla oblongata, the pons, and the midbrain – which together make up the brainstem – and the thalamus.
The cochlear nucleus¶
The auditory nerve penetrates the skull and forms a first relay station at the level of the cochlear nuclei in the pons. The incoming fibers are distributed in a tonotopic, manner: those that come from the apex of the cochlea (low frequencies) remain at the surface of the cochlear nuclei, whereas those from the base (high frequencies) penetrate deeper into the nuclei. Each fiber divides into a “V” shape with two branches: one ascending to the anteroventral (AVCN) and posteroventral (PVCN) nuclei and another descending to the dorsal cochlear nuclei (DCN:
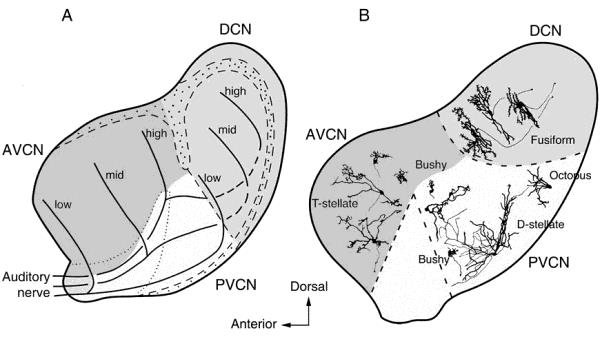
Fig. 61 A sagittal section of the cat cochlear nucleus showing its three divisions.¶
Figure 1.6 from {Pickles, 2015}, which took it in turn from Osen (1969) and Oertel (1991).
As seen in The ascending auditory pathways 1, projections from both the dorsal and anteroventral nuclei continue on to the primary auditory pathway, but only the posteroventral nuclei project to the non-primary auditory pathway.
The primary auditory pathway¶
The cochlear nuclei decode intensity, duration (long or short sounds) and the start and end of a sound, and maintain and transmit the frequency analysis carried out at the level of the cochlea.
Dorsal cochlear nucleus¶
Fusiform neurons¶
{Tzounopoulos, 2008}
The DCN is an auditory brainstem nucleus involved at the earliest stages of sensory processing and is believed to play a key role in orientation toward sounds of interest and/or suppression of responses to expected body-generated sounds (Shore & Zhou, 2006; Sutherland, Glendenning & Masterton, 1998; Young & Davis, 2001). DCN fusiform neurons receive input from the auditory nerve and from a system of parallel fibers carrying multimodal input, including proprioceptive signals encoding the position of the ears, as well as input from the vocal tract perhaps signaling suppression of body-generated sounds or vocal feedback (see Figure 1). DCN parallel fibers also activate inhibitory glycinergic cartwheel cells, which provide feed-forward inhibition to fusiform cells. The complex response of fusiform cells to auditory signals is shaped by feed-forward inhibition from additional cell types, the vertical cells of the deep layer (see Figure 1) and D-stellate cells of the ventral cochlear nucleus (Young & Davis, 2001).
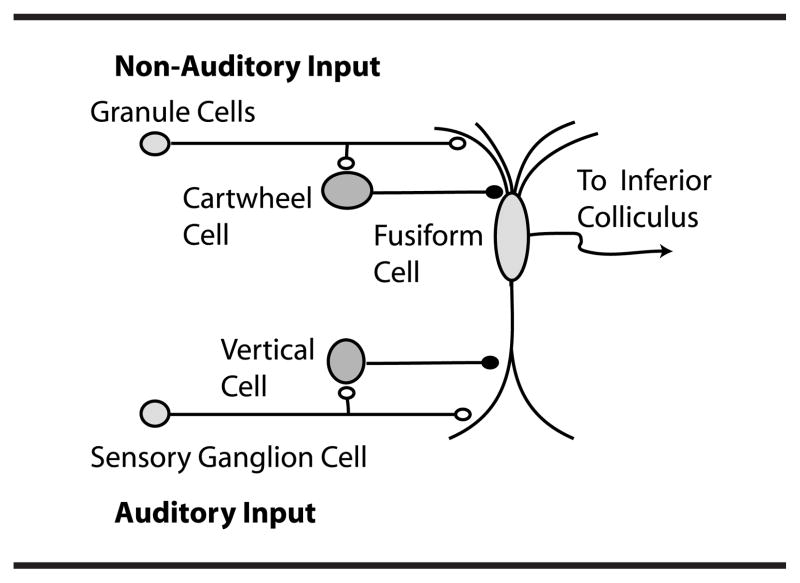
Fig. 62 Dorsal cochlear nucleus (DCN) circuitry¶
Figure 1 of {Tzounopoulos, 2008}
{Shore and Martel, 2018}
the DCN is thought to adjust perception by incorporating predictive signals from the somatosensory system (Bell, Han, Sugawara, & Grant, 1997; Nelson, 2004). Internally generated sounds that arise from movement, as in head orientation, vocalization or chewing, recruit the somatosensory system, producing near-coincident auditory and somatosensory inputs to DCN. The circuit adaptively adjusts fusiform-cell output to amplify external (unpredicted) auditory cues while subtracting internally generated sounds. This process likely involves voluntary motor commands processed by the PN, which, in addition to relaying cortical motor efferent projections to the cerebellum, sends projections to the GCD (Babalian, 2005; Ohlrogge et al., 2001), thereby routing motor information to the DCN. Thus, the incorporation of motor as well as sensory information into this circuit may be fundamental for sound localization in the vertical plane (Davis, Ramachandran, & May, 2003; May, 2000) and for suppression of body-generated sounds, in addition to the acknowledged role of the trigeminal brainstem nucleus (Requarth & Sawtell, 2011; Singla, Dempsey, Warren, Enikolopov, & Sawtell, 2017).
Anteroventral cochlear nucleus¶
Superior olivary complex¶
{Felix and Magnusson, 2016, neuro}
The superior paraolivary nucleus (SPON) is a prominent structure in the mammalian auditory brainstem with a proposed role in encoding transient broadband sounds such as vocalized utterances. … we measured evoked excitatory postsynaptic currents (EPSCs) in the SPON originating from the intermediate acoustic stria and compared them with the properties of EPSCs in the lateral superior olive (LSO) originating from the ventral acoustic stria during auditory development from postnatal day 5 to 22 in mice.
Before hearing onset, EPSCs in the SPON and LSO are very similar in size and kinetics.
After the onset of hearing, SPON excitation is refined to extremely few (2:1) fibers, with each strengthened by an increase in release probability, yielding fast and strong EPSCs. LSO excitation is recruited from more fibers (5:1), resulting in strong EPSCs with a comparatively broader stimulus-response range after hearing onset. Evoked SPON excitation is comparatively weaker than evoked LSO excitation, likely due to a larger fraction of postsynaptic GluR2-containing Ca2+-impermeable AMPA receptors after hearing onset. Taken together, SPON excitation develops synaptic properties that are suited for transmitting single events with high temporal reliability and the strong, dynamic LSO excitation is compatible with high rate-level sensitivity. Thus, the excitatory input pathways to the SPON and LSO mature to support different decoding strategies of respective coarse temporal and sound intensity information at the brainstem level.
Lateral lemniscus¶
Inferior colliculus¶
Medial geniculate nucleus¶
Other¶
{Zoefel and VanRullen, 2016, #8293}
We presume that entrainment to low-level features of speech sound occurs relatively early in the auditory pathway (i.e., somewhere between cochlea and primary auditory cortex, including the latter; Davis and Johnsrude, 2003 and Lakatos et al., 2005), whereas entrainment to high-level features occurs beyond primary auditory cortex (Uppenkamp et al., 2006).
The non-primary auditory pathway¶
Posteroventral cochlear nucleus¶
Reticular formation¶
http://www.cochlea.eu/en/auditory-brain
In the reticular pathway of the brainstem and the mesencephalus, several synapses occur. It’s here that the auditory information is integrated with all the other sensory modalities to be ‘triaged’ into which has the highest priority at any given moment. In other words, the reticular pathways participate with the wake and the motivation centres in selectioning the information that should be treated as priority by the brain.
Non-specific thalamus¶
Decussation¶
Descending information¶
Reflexes¶
Summary¶
References¶
Schnupp, J. W. H. (2013). Coding in the auditory system. In R. Q. Quiroga & S. Panzeri (Eds.), Principles of Neural Coding (pp. 179-204). CRC Press.
Netter’s Atlas of Human Neuroscience. Author:David L. Felten, MD, PhD … Chapter:Sensory Systems Page:228; http://www.amazon.com/The-Netter-Collection-Medical-Illustrations/dp/1416063846
Tzounopoulos, Thanos. “Mechanisms of Synaptic Plasticity in the Dorsal Cochlear Nucleus: Plasticity-Induced Changes That Could Underlie Tinnitus.” {American Journal of Audiology} 17.2 (2008): S170-75.
Felix, Richard A., and Anna K. Magnusson. “Development of Excitatory Synaptic Transmission to the Superior Paraolivary and Lateral Superior Olivary Nuclei Optimizes Differential Decoding Strategies.” Neuroscience 334 (2016): 1-12.
Pickles, J. O. 2015. Auditory pathways: anatomy and physiology. In Handbook of Clinical Neurology, eds. Aminoff, M. J., Boller, F., Swaab, D. F., pp. 3-25. Elsevier
Tzounopoulos, Thanos. “Mechanisms of Synaptic Plasticity in the Dorsal Cochlear Nucleus: Plasticity-Induced Changes That Could Underlie Tinnitus.” American Journal of Audiology 17.2 (2008): S170-75.
Shore, S. E., Martel, D. T. 2018. Multimodal inputs to the cochlear nucleus and their role in the generation of tinnitus. In The Oxford Handbook of the Auditory Brainstem, ed. Kandler, K., Oxford University Press
Endnotes¶
The next topic¶
Come to class having read Cortex and answered the questions.
Last edited Aug 22, 2023